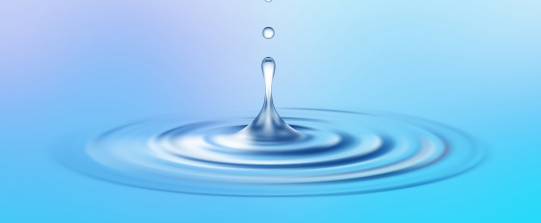
DOWNLOAD
DATE
Contact
Government agencies, water and wastewater utilities, and engineering and mining companies are seeking to optimize water supply, decrease water consumption, and recycle water needed for sustainable production of critical metals and minerals. Recently, Arthur D. Little (ADL) took a deep dive into mining in Chile and studied the actions the industry there took to address the country’s water scarcity issues. Other parts of the world struggle with similar obstacles and may find guidance in this Viewpoint useful to help overcome them.
Mining is one of the main drivers of the Chilean economy; most of the country’s mining sites are located in the mountainous, extremely dry north region. In 2021, the International Trade Administration (ITA) reports that the mining sector contributed US $317 billion to the Chilean economy, which equaled about 15% of Chile’s GDP. Mining exports were over 62% of the country’s total exports.
The mining process is complex and often requires substantial amounts of water. Falling ore grades and growing demand for metals like copper and lithium push mining projects into increasingly remote locations. These metals exist in harsh, arid, high plain desert locations that may also be characterized by severe water scarcity. Australia, Saudi Arabia, Morocco, and parts of Africa experience harsh conditions similar to Chile’s, which hamper successful mining operations.
The global economy is contending with energy transition and decarbonization, population growth, and upheaval in supply chains, all of which rely on increasing amounts of raw materials including minerals and metals. The demand for recycling, sustainable water supply methods, and strategies to lower water consumption have dictated new complex requirements to technology, energy suppliers, funding mechanisms, regulations, operational models, and public-private partnerships. We use examples from Chile’s mining industry to describe how the above entities addressed the complex issue of supplying water for its operations.
ABUNDANT COPPER & LITHIUM, BUT NO WATER
The ITA reports that about a third of the world’s copper is produced in Chile, which is also the world’s second-biggest producer of lithium with approximately a quarter of the global share. Chile also has the largest reserves of copper and lithium (200 million metric tons and 9.2 million metric tons, respectively) in the world. Its supply of these metals is more than twice that of Australia, which has the world’s second largest supply of these extremely in-demand metals required for green economy.
Most of the country’s copper and lithium reserves are located in Antofagasta, Atacama, and Tarapacá — all very arid regions in northern Chile. Atacama is one of the driest places on the planet, made up of sand, stone, and salt lakes.
Water is used during mining activities at multiple stages. In copper mining — primarily during the concentration steps of copper ores, which includes comminution of the mineral, followed by flotation, classification, and thickening — approximately 90 cubic meters of water is needed to produce one ton of copper. In lithium mining, the lithium is dissolved in a brine aquifer below the salt crust. To extract it, it is necessary to pump water into salt beds to transport it to the surface. The mixture of salt, minerals, and water sits on vast areas of land for about a year, waiting for the water to evaporate so that it can be used for production. Approximately 2,000 cubic meters of water is needed to produce one ton of lithium. Thus, the mining operations in those regions need to desalinate and deliver a substantial share of needed water from 150+ kilometers from the sea and at heights of 2,000-4,000 meters.
Chile’s water scarcity can be explained by geography; when regions are situated between two mountain ranges of sufficient height, they prevent moisture advection from the surrounding Pacific or Atlantic Oceans, resulting in a two-sided rain shadow. Existing natural groundwater sources in the country are insufficient even to meet demands of agriculture and the local population. Thus, water for mining operations in those regions must be desalinated and delivered across long distances and over great heights. Figure 1 shows the supply and demand for fresh water in Chile by region.
SEAWATER DESALINATION & DELIVERY
Mining companies, utilities, and engineering companies have focused intently on developing seawater desalination and transportation infrastructure with support from the Chilean government in a variety of alliances.
In 2008, the country’s first seawater desalination plant was commissioned and designed to supply mining sites with water. By 2021, nine plants and three seawater impulsion systems were operational. The system brings seawater into the desalination plant, uses a reverse osmosis process to filter out the dissolved mineral and biological residue, and circulates extracted brine and other substances back into the ocean. The resulting desalinated water is then pumped into the mine through two pipelines, both 42 inches in diameter, which are located 3,200 meters above sea level. Four high-pressure pumping stations, a reservoir at the mine, and high-voltage electricity infrastructure are needed to operate the system.
Future projects will increase the current desalination capacity by 130% by 2031, when the Antofagasta region will have the largest number of desalination plants in the country, supplying 66% of the copper industry’s consumption in that region (see Figure 2).
OPERATIONAL MODELS & CONTRACT TYPES
Mining in Chile is very profitable, so most water supply projects are funded and organized by mining companies to benefit their operations. Most often, mining companies and government entities collaborate and develop public-private partnerships with engineering procurement construction (EPC) or engineering procurement and construction management (EPCM) companies that specialize in water desalination. Partnerships can take a variety of approaches to planning, funding, designing, constructing, and operating water supply projects. Here are two examples:
-
BHP Group Limited teamed with Tedagua, a Spanish water treatment company, and Saipem, an Italian engineering company, to develop a water supply project for the Spence Growth Option (SGO) project at the Spence copper mine (see Figure 3). The plant uses reverse osmosis technology with a production capacity of 1,000 liters per second. Construction projects include a seawater intake works and the marine intake and outfall pipes, as well as a 154-kilometer-long steel pipeline with a diameter of 36 inches. Three pumping stations will pump desalinated water to the mine’s facilities, overcoming slopes of over 2,000 meters. A 66-kilovolt, 75-kilometer-long overhead power line will be built to supply the plant with energy. The three organizations assume responsibility for financing, design, construction, operations, and maintenance.
-
Codelco, a Chilean state-owned copper mining company, has signed a BOOT (build, own, operate, transfer) contract with Marubeni Corporation, a Japanese investment company and Transelec, the leading power transmission company in Chile. This consortium will build, own, and operate the project. The RT Sulfuros plant’s planned capacity is 1,956 liters per second (see Figure 4). It will be complemented by a 160-kilometer-long water delivery line to the Radomiro Tomic mine, located 3,000 meters above sea level, and five pumping stations, to be covered by two 110-kilovolt and 220-kilovolt high-voltage lines. This modern desalination plant will produce and transfer water through a drive line with a buried steel pipe that will prevent visual contamination of the landscape and allow unencumbered transit for people and wildlife.
Other business models exist where mining companies give design and construction orders to subcontractors who plan, fund, and operate desalination plants. For example, Kansas, USA-based Black & Veatch, an EPC and consulting company, was selected by BHP to design desalination facilities for the Escondida Water Supply project.
WATER CONSUMPTION DECREASE & REUSAGE
In addition to water desalination and supply measures, leading mining companies throughout the world work to develop new technologies to reduce water usage in mining and beneficiation operations. Many mining industry players use their Chilean deposit and production assets for testing and piloting these technologies and employ those technologies to further decrease water consumption. Included among such technologies are:
-
Magnetic resonance (MR) analysis. Determination of high-grade ore portions can be performed using MR analysis at the mining site. In modern high-performance sorting systems, such an analysis is performed in real time, measuring the resonance of radio impulses from ore batches. Ore that falls below the specified limit is immediately transported to the dump and is discarded. This can be compared to a medical MRI — if the results do not confirm the need for surgery, it will not be scheduled. Using this technology significantly reduces unnecessary processing volumes, reduces costs, and provides proportional water savings.
-
Coarser particle flotation. Crushed into powder, the ore is fed to flotation units filled with a water solution and added foaming agents. Under pressure from below, air is supplied to form bubbles, to which particles of the mineral necessary for further processing adhere. This foam rises to the top and is separated and transferred to the next stage of processing. Unnecessary materials remain below and are removed.
Specialists have developed various options for flotation technologies that allow two to three times larger granules to float to the surface than was previously possible. The ore obtained in this way contains large particles, sticks together less, is more easily shed of water, and can be recycled in a larger volume. As a result, water consumption is reduced by more than 20%. In addition, the recovery rate is increased, the energy required for grinding is reduced, and the waste is a drier material that can be stacked, which reduces the area required for tailings.
-
Dry disposal. When used in conjunction with coarser particle flotation, this technology can reduce water consumption by more than 50%. Traditional tailings dams contain waste with a high water content. New dry disposal technologies allow for pressing and filtering waste, removing water that is in turn recycled to the plant. This requires additional costs, although in the mountainous deserts of Chile, where water costs $5 per cubic meter, the expense is compensated by water savings.
MAKING DESALINATION WASTE USEFUL
What happens to the concentrated brine produced by seawater desalination on multiple plants? Unfortunately, waste from the process is dumped back into the sea, using costly, energy-consuming pumping systems as well as damaging marine ecosystems. However, researchers at Massachusetts Institute of Technology (MIT) recently arrived at an alternative solution.
They identified a process that converts waste material into useful chemicals — including chemicals that can make the desalination process itself more efficient. This approach can be used to produce sodium hydroxide, among other products. Otherwise known as caustic soda, this compound can pretreat seawater entering the desalination plant. Caustic soda changes the acidity of the water, helping prevent fouling the membranes used to filter the saltwater — a major cause of interruptions and failures in desalination plants that use reverse osmosis. Because desalination plants need large quantities of the compound, it must be procured and transported from chemical plants, but making caustic soda on-site could prove advantageous. The amount needed by desalination plants themselves is far less than what could be produced from the brine, so any excess could be marketed and sold.
Hydrochloric acid is another important chemical used by desalination plants. Hydrochloric acid can be made on-site from waste brine using established chemical processing methods. It can be used to clean parts of the desalination plant, but is also widely used in chemical production and as a source of hydrogen. The method includes nanofiltration to remove undesirable compounds, followed by one or more electrodialysis stages to produce the desired end product.
The world currently produces more than 100 billion liters of water a day from desalination, which creates a similar volume of concentrated brine. Converting the brine can be both economically and ecologically beneficial, especially as desalination continues to increase rapidly around the world.
Conclusion
FUTURE APPLICATIONS
Mankind has invented many ways to produce, deliver, and use water more economically for mining in the most remote and arid places on the planet. Examining the steps taken by the various stakeholders in Chile reveals actions that can be replicated elsewhere:
-
Large plants used reverse osmosis desalination to produce thousands of liters of fresh water per second.
-
Extensive infrastructure, which included pumping facilities, pipelines, and water storage facilities, brought water across great distances and over great heights.
-
New mining technologies were developed to decrease water consumption and safely reuse it when applicable.
These projects require cooperation among people, organizations, and nations; substantial investments of time and money; and mindful planning that considers the full range of interconnected, long-term consequences, while ensuring the resilience of our planet’s fragile ecosystem.