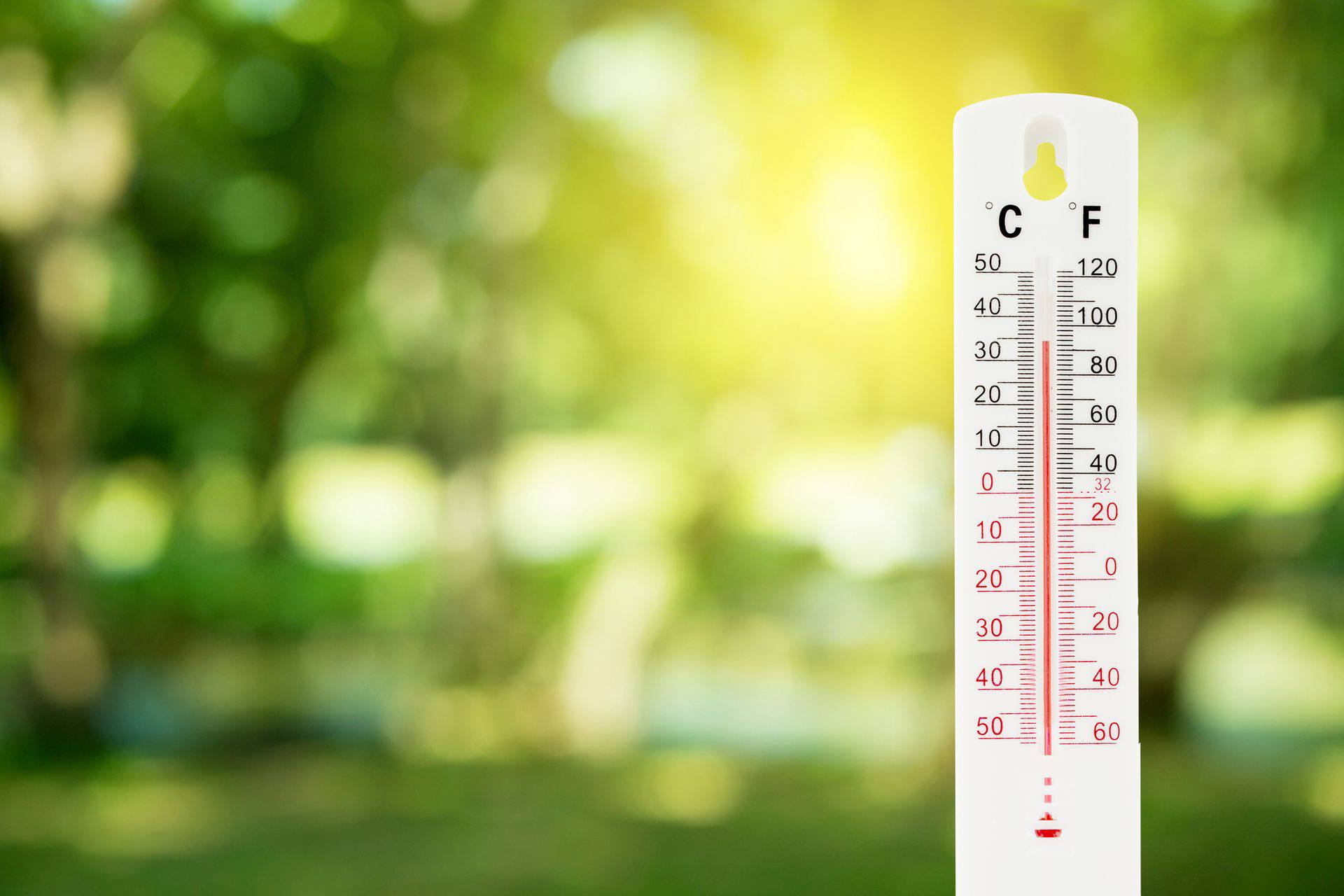
DOWNLOAD
DATE
Contact
We often associate reducing carbon emissions with buying “green” electric power, switching to electric cars, or reducing, reusing, or recycling waste. Indeed, renewable power jumped to 29% market share of global power generation last year, up from 27% in 2019, according to IEA. Yet, only 10% of heat, half of the world’s energy consumption, comes from renewables. Options in decarbonizing heat are more complex and expensive than reducing carbon in power generation. However, heat decarbonization technologies are becoming more attractive. So, on one hand, decarbonization of heat is difficult for energy companies active in heat production; on the other hand, it opens up business opportunities in providing no-carbon heating solutions to customers. In this Viewpoint, we look at solutions and markets for heat decarbonization and explore strategic options for energy utilities.
Heat’s huge carbon footprint
Heat represents almost 75% of industrial energy demand and 63% of residential energy. Carbon-based fuels account for 90% of heating energy; 60% comes from carbon-intensive coal and oil (see figure below). Residential areas have myriad energy sources. In countries with high heat demand, for example, fossil energy carriers dominate, while in warmer zones, cooling needs require electricity. Large cities and communities with district heating utilize various carbon-intensive (coal and gas) and renewable sources (biomass and waste heat). Often, this allocation depends on a country’s natural resources and circular economy. For instance, in Russia, district heating with fossil fuels accounts for more than 20% of total energy, while in Sweden nearly 15% of district heating operates with about 70% share of renewables. While district heating can be a residential option, it is harder to supply industrial companies. Industrial heat requirements range from up to 150°C often continuously, while district heating operates seasonally at up to 130°C (in winter). Today, only up to 10% of industrial energy consumption comes from renewable sources (e.g., biomass, biogas). (Source: IEA)
Power-to-heat: technology of choice to decarbonize
Decarbonization of heat is a much bigger challenge than producing carbon-free electric power. Almost all carbon-neutral energy stems from electric power, be it hydro, solar, or wind. But, according to IEA, only 6% of all global primary energy supply is biomass, biogas, or waste heat, which allows for direct use for heating. Since sources of bioenergy are limited, most carbon-free heat must come via renewable or nuclear electric power. Due to its versatility, transportability, and ability to become carbon-free, electric power is the most valuable energy form. The technology of choice to decarbonize heat without using biomass is the heat pump – due to its high efficiency using renewable power. A heat pump makes about 3.5 kWh heat for residential purposes from 1 kWh of electric power by leveraging low temperatures.
Residential heating sparks growth
In Europe, about 15 million heat pumps are installed, covering about a 13% share of all residential buildings. (Source: EHPA) Most frontrunners with high shares of heat pumps are characterized by low power prices based on hydro or nuclear power, such as Switzerland, France, Norway, and Sweden.
While heat pumps still have a niche position in many markets, increased growth is coming. By 2025, sales are expected to rise significantly due to tighter carbon regulation, especially carbon tax on oil and gas and energy efficiency targets for new buildings. Biomass will stagnate, while solar thermal gets an extra boost.
The number one growth driver is the housing new build rate. In new or energetically revamped homes, built according to recent energy standards, heat pumps have a clear financial advantage. They can be utilized in older homes as well if some energy efficiency basics are fulfilled (e.g., insulating glass windows). Some governments offer subsidies; for example, there are considerable investment grants in Germany to foster energetic refurbishment and heat pumps. But growth of power-to-heat doesn’t come easy. In some markets, electric power prices are high even with existing heat pump tariffs. Ground-source heat pumps have a higher efficiency than air-source heat pumps but require costly ground collectors or ground probe drilling. Backup heat and hot water supply can come on top of this investment.
Decarbonizing district heating
District heating is common worldwide, especially in countries with lower temps. This efficient method, mostly occurring in large cities, can supply heat to a vast customer base in close vicinity using combined power and heat synergies, waste heat recovery, and large thermal power plants. Recently, local heating grids are springing up in efforts to supply a neighborhood or small district. Low-temp grids, however, are rarely used as an option to avoid loss of thermal energy. Here, grid temperature is reduced to up to 50°C, enough to supply heat pumps at customer sites and produce individual high-temp heat, allowing for decarbonization of heat by integrating heat pumps efficiently. Most district heating systems are operated with fossil fuels or – to a lower extent – with biomass or waste.
District heating has a twofold relation to decarbonization: (1) it must be decarbonized; (2) a decarbonized heat grid can connect more buildings to supply clean heating. District heating as a strategy decarbonizes cities in an efficient, (relatively) easy, and cost-efficient way for a large number of end customers – and it helps win new customers.
Industrial heat challenges
Heat enables manufacturing processes in paper, food, chemical, and many other industries. Many industries have specific heat requirements, mainly high-temp grades and a high-temp quality to ensure conformance with quality standards. According to the European Commission, half of European industrial heat demand expected for 2030 is above 500°C. Waste heat as a byproduct, or as remaining energy that cannot be used for the industrial process, can be captured via a heat exchanger and transported to another heat sink (e.g., neighboring firms for room heating or warm water). Moreover, cool air or water generated as a byproduct from a neighboring heat pump user can be reused. Thus, warehousing with heat demand can create mutual benefits with the cooling demand of a neighboring food company.
Power-to-heat technologies for producing carbon-free, hightemp heat include electrode boilers and high-temp heat pumps with maximum temperatures of 240°C and 200°C, respectively. Current low gas prices delay industrial deployment of power-to-heat solutions in this temperature level. Not all heat requirements can be fulfilled by power-to-heat, since high temps above 240°C are not commercially available. Instead, other carbon-free energy sources like renewable power or green hydrogen and green gas must be used.
Green hydrogen in heating
Green hydrogen produced via electrolysis from renewable power, mainly wind and solar, can be used for heating either pure in fuel cells or dedicated hydrogen boilers, or as synthetic green methane made from hydrogen and CO2 captured from the air. The hydrogen source and the conversion of hydrogen to heat both significantly impact cost (see figure below).
From a consumer point of view, using hydrogen can be more expensive than a heat pump, including an auxiliary boiler for the coldest hours of the year. However, hydrogen might be justified when heat pumps are inefficient (e.g., in city quarters with many old buildings where insulation is not possible or allowed). From a national/municipal point of view, hydrogen could be preferred for several reasons: existing gas grids/storage can be reused with minor investments in upgrading to hydrogen; imported hydrogen could avoid bottlenecks in renewable power for end customer; when adding hydrogen natural gas, customers have no change issues with new boilers, and so on. Distributing pure hydrogen through gas grids is not yet practiced; however, it is currently being tested (e.g., by gas grid operators in the UK and Germany).
Hydrogen comes into play for large-scale, long-term storage of renewable power – usually seasonal when wind or solar are unavailable in sufficient capacities. However, dedicated turbines or fuel cells are needed to make use of it. If only heat is required, hydrogen can be burned in hydrogen-ready boilers. In district heating, hydrogen – combined with solar photovoltaics (PV) and electrolysis – could provide energy autonomy locally; however, this comes at a high CAPEX/OPEX level.
Options for cities
Supplying all fossil-heated buildings via heat pumps is possible but not necessarily optimal. When we consider various building types, heat supply, utilization purposes, building ages, insulation, and so forth, clearly there are many different configurations of a heat infrastructure for a town or city quarter. All that calls for a systematic, holistic planning approach for energy. There are at least three basic configurations for a decarbonized city/quarter heating solution (see figure below):
- Green district heat. Utilizes existing heat grids and plants; however, operates carbon-free. Depending on heat requirements, biomass, green gas, or large heat pumps are suitable solutions. Final solution depends on required temps and available infrastructure/fuels. The key advantage is time to market. By switching fuel in a heat plant, thousands of homes/offices can be supplied with carbon-free heat. Also, grid extensions are possible with limited incremental investment in more heat pipes. Customers do not need to invest, switch heating behavior, or make changes to their homes. Overall, this is a smart solution; however, it requires an existing heat grid or a heat grid new build, which needs high commitment from potential end users and the municipality.
- Green gas. Reuses existing gas grid and customer gas boilers; however, 100% green gas is either hydrogen from renewable power, synthetic gas from renewable hydrogen, or biogas. Biogas is a short-term solution, allowing for switching to hydrogen or synthetic carbon-free gas later. It is also fast to implement and leaves the customer with the choice of when and how to switch (at least for homes with dedicated gas boiler).
- Heat pump. Requires building owner’s decision to make significant investment in equipment, thermal insulation, and new radiators. For apartment buildings, a dedicated hot water supply at minimum temperatures requires an extra heating source. The speed at which the transformation of a city/quarter can take place with heat pumps is low; it depends almost completely on the single homeowner’s decision. However, in new builds, recent energy efficiency requirements make heat pumps the solution of choice.+
What’s on the horizon for energy utilities?
Decarbonization calls for a massive transformation of the still-dominating fossil fuel–based heat economy. This will greatly impact most energy utilities: from gas downstream to power and heat generation to energy service providers. At first glance, power-to-heat creates both positive and negative effects, depending on the business. As an energy supplier, reducing carbon means less demand for fossil gas (or oil) but more demand for electric power. Even stronger is the impact on gas-based heat contracting companies, which might lose customers over time. Plus, due to the huge amount of carbon emissions from heat generation, energy companies will face a negative impact on their core business (especially gas or traditional heat suppliers) but also can identify a wide range of customer solutions, bringing in new business. With myriad carbon-free heating solutions, however, companies can tap multiple new revenue sources. For example, replacing today’s oil or gas heating via grid or solar-powered heat pumps could bring additional revenues and higher customer retention through leasing/contracting.
What should energy utilities do?
The impact of the energy transition has been identified by most utilities some years ago, considering the growth of renewable power generation, energy storage, energy efficiency policies, smart grid, and smart home/building technologies. Strategies and initiatives around these topics were and still are the answer. Comprehensive, radical decarbonization toward zero carbon will have a tremendous impact on heat emissions within the next 25 years; however, this is an unprecedented challenge.
A key strategy is to select the right ambition level that fits best to the market environment and the utility’s purpose:
- Wait and see. This is a reactive approach, where action is taken when regulations require it or a clear, lasting change in market conditions is obvious. This might mean staying the course with gas sales and existing heat grid, as long as regulatory requirements are not forcing change.
- Selective innovation. Whenever a new technology becomes mature, or policy regulation change allows, innovative “point” solutions are launched in the market. Most innovations in heat are around heat pumps and local solar power. They do not change the business model and rely on limited changes and thus have limited risks.
- Radical innovation. This happens when a whole system or business model undergoes a fundamental change. In the heat world, examples are switching from gas grid to hydrogen; phasing out gas and moving to all-electric heat solutions based on heat pumps; or decentralizing heat/ power supply in local grids, quarter/community solutions, or similar new shared business models.
Another approach to decarbonization is to consider the degree of uncertainty: what kind of solution and technology will be successful? This approach contains four steps that range from low-risk moves to a fundamental redesign of the current business model and infrastructure:
- “No regret” moves are possible in the retail business; for example, helping customers to install heat pumps and manage necessary changes in radiators or building insulation.
- An incremental move would be to retrofit district heat plants from fossil fuel to biomass and/or heat pumps, including an extension of the heat grid into new districts of a city.
- More radical moves are semiautonomous district solutions, which combine local power and hydrogen generation (PV, combined heat and power, biogas) with heat pumps, storage, and EV charging for part of a city/region. In a world with district solutions, a utility can take different roles and business models: from providing selected services and infrastructure (e.g., heat pumps, power, and gas grid) to acting as a holistic district energy manager that orchestrates the “prosumers.”
- A fundamental move is the transition from natural gas to 100% green hydrogen, with several strategic advantages: improves security of supply by adding second energy carrier to electric power; reduces risk of power grid bottlenecks, or even outages in case of low renewable power availability; could supply industry with feedstock and high-temp heat; and reduces costly upgrades of district heating plants with heat pumps. The risk lies in investment allocation to infrastructure in favor of long-term, continued operation of pipeline capacities in demarcation from district heating and the power grid. Profitability depends largely on future price competitiveness of green hydrogen in heat supply.
All four options require dimensioned budgets and risk-balancing moves such as partnering. Overall, energy utilities should implement a holistic heat strategy, which is key to long-term market position, growth, and profitability.