Is steel scrap the new gold?

Steel production is responsible for 7%[1] of global carbon dioxide (CO2) emissions, underscoring the urgency of reducing its carbon footprint to meet net zero targets. Regulators are intensifying their efforts; for instance, Europe aims to cut CO2 steel production emissions by nearly 25% by 2030.
Steel is integral to the global economy, playing a crucial role in the construction, automotive, and industrial machinery industries. It is also essential for green technologies such as wind turbines, electric vehicles, and advanced manufacturing processes. Despite volatility, steel demand is projected to rise, making its decarbonization pivotal for achieving a greener economy.
Two key technologies promise a more sustainable future for steel production: direct reduced iron (DRI) for primary steel and electric arc furnaces (EAFs) for secondary production, which reuse scrap steel. Deploying these green steel technologies is crucial for reducing emissions and replacing traditional, coal-fired blast furnaces.
However, successfully building and running DRI and EAF steel mills requires substantial investments, a supply of competitively priced green energy, and — the primary lever for EAF — acquiring sufficient scrap steel. In 2022, out of the 1,885 metric tons (Mt) of crude steel production, 1,340 Mt were produced using blast furnaces (around two tons of CO2 per ton of liquid metal), which should be replaced by DRI and EAF. EAF production was 538 Mt in the same period. This will drive large-scale demand growth in the scrap market. Scarcity will probably drive prices higher, particularly for clean scrap generated by manufacturing processes.
To secure their futures and meet the need for this vital material, steelmakers must transform to create more circular economies to secure and effectively use scrap. They need to move from digging in the ground for materials to digging in recycling sources. That requires closer collaboration with recyclers and manufacturers across the ecosystem that create scrap as a byproduct of their activities, such as automotive manufacturers, to collect sufficient volumes to power their new mills.
These changes are prompting steel producers to look globally for scrap, sourcing this new “gold” from Africa, China, Latin America, Europe, and North America. This expansion necessitates new strategies and operational models.
How can steel producers manage the green transition, and what are the lessons for other sectors as economies become more sustainable and circular?
MEETING THE GLOBAL IMPERATIVE TO DECARBONIZE STEEL
REGULATORY PRESSURES
Traditional steel production methods involve blast furnaces that use coking coal as fuel and inevitably produce large amounts of CO2. Around 85% of steel production was powered by fossil fuels in 2022, according to the World Economic Forum.[2] While efforts are ongoing to reduce or capture emissions, such as by carbon capture and storage (CCS), large-scale deployment remains limited because of economic, technical, and infrastructural challenges. Many projects are still at the proof-of-concept stage, such as ArcelorMittal’s Steelanol Project in Belgium, which aims to capture CO2 from steel production and convert it into ethanol through a biological process.
Meanwhile, the regulatory clock is ticking. Annual emissions from steel production represent 5% of the EU’s total CO2. This has led EU regulators to target reducing emissions from 191 Mt in 2021 to 150 Mt by 2030. Individual firms have also set their own targets; for example, US Steel aims to reduce greenhouse gas emissions intensity by 20% by 2030 and achieve net zero emissions by 2050. Regulatory change is being driven by a combination of targets, carbon taxes (including the EU’s Carbon Border Adjustment Mechanism [CBAM], which aims to ensure imported materials such as steel are produced sustainably), and national/international subsidies for the switch to cleaner technologies such as DRI and EAF.
CUSTOMER PRESSURES
Major customers are also increasingly demanding low-emission steel, driven by their own sustainability targets. For example, leading US automotive manufacturers such as Ford and General Motors have joined the First Movers Coalition, a global group committed to decarbonizing supply chains, including steel.
THE IMPORTANCE OF TECHNOLOGY INNOVATION
Together, these regulatory and customer pressures are driving a need for change in the steel industry, which is manifested in a shift away from emissions-heavy blast furnaces to cleaner technologies that rely on three elements:
- Green energy
- Hydrogen
- Sufficient supplies of scrap
DRI AND EAF
DRI furnaces replace the coking coal traditionally used in the iron ore reduction process with gas or hydrogen to produce directly reduced (liquid) iron. This is then fed into an EAF, along with scrap steel, at a ratio of at least 50% scrap. This combination is melted to form liquid steel. It is then cast and rolled to transform the steel into coils or plates.
Emissions are considerably reduced, particularly if green feedstock (such as green hydrogen) replaces coking coal and the electricity used within the EAF is from renewable sources. However, the cost of producing liquid metal will increase without access to cheap, decarbonized energy to power these processes.
Demonstrating the potential of this green steel, steel producer SSAB, iron ore miner LKAB, and energy company Vattenfall collaborated to create HYBRIT (Hydrogen Breakthrough Ironmaking Technology), a fossil-free iron and steelmaking technology. In June 2021, the three companies showcased the world’s first hydrogen-reduced sponge iron, which was then used to produce steel provided to Volvo. Volume production by SSAB is scheduled to begin in 2026.
THE CHALLENGES TO THE PRODUCTION OF GREEN STEEL
Five challenges face the steel industry as it aims to transition from old-style blast furnaces to cleaner DRI-EAF steel production: technological maturity, growing CAPEX/OPEX investment, securing green energy, securing sufficient scrap, and the need for new skills/capabilities.
1. TECHNOLOGY MATURITY
EAF technology is fully mature and already makes up a substantial proportion of global steel production volumes. It is currently being extended to make new steel types. However, it requires a combination of scrap and primary steel from DRI plants, replacing inputs from blast furnaces. Yet, DRI technology is currently at the developmental stage, with many existing installations relying on natural gas rather than green fuels such as hydrogen. While significant investments have been announced in DRI (over €10 billion in Europe alone), no DRI facilities of 2.5 Mt that consume hydrogen have yet been built. Therefore, scaling green DRI pilots into mature installations is critical to reducing emissions and ensuring a green future for steel.
2. GROWING CAPEX AND OPEX INVESTMENT
In mature markets, many previous investments focused on process improvements or increasing capacity/quality. Business cases were, therefore, relatively simple to calculate based on projected market demand. Securing investment in green steel projects is much more complex, as it requires assumptions on CO2 prices, energy costs, and planned regulations/carbon taxes. According to Eurofer, meeting the EU’s 2030 targets requires producers to invest an estimated €85 billion — €31 billion in CAPEX and €54 billion in OPEX — of which €15-17 billion has been announced. This is leading to heavy reliance on state subsidies to fund new plants around the world, including:
- €850 million from France to ArcelorMittal
- €2 billion from the German state to ThyssenKrupp
- £500 million from the UK government to Tata Steel
- US $500 million each from the US Department of Energy to steelmakers Cleveland-Cliffs and SSAB for green steel plants
3. SECURING GREEN ENERGY
Producers must source a continuous green energy supply for DRI and EAF facilities. Consequently, replacing blast furnaces with DRI-EAF means energy will make up a much higher percentage of running costs than iron ore based on current market conditions. Securing these energy supplies at competitive prices is vital for success:
- Green and blue hydrogen for DRI: Competition is growing for (currently limited) green and blue hydrogen supplies, which require renewable energy for production and carbon capture technology, respectively. A significant reduction in the price of hydrogen is necessary to make DRI cost competitive.
- Green electricity for EAF: Given the need for constant electricity availability to power EAFs, hydroelectric and nuclear power are the most appropriate sources, rather than intermittent solar or wind. However, the availability of such sources varies considerably by country and region, again pushing up costs and handing advantages to producers located near such energy sources.
Recently announced European DRI and EAF projects are estimated to require 60 gigawatt hours (GWh) of green energy annually, covering both the direct supply of green electricity and the green energy required to produce hydrogen through electrolysis.
Investments are also being made in new sites that benefit from access to renewable energy and green hydrogen. Vulcan Green Steel (VGS) has begun construction of a greenfield steel complex in Duqm, Oman. When production begins in 2027, it will run on 100% renewable wind and solar energy and incorporate green hydrogen once it becomes available.
4. SECURING SUFFICIENT SCRAP
Scrap steel is essential for EAFs, in which it is melted alongside liquid iron from DRI. It comes from three main sources (see Figure 1):
- Internal “clean” steel mill scrap that is traditionally consumed by the producer
- Clean scrap from downstream players that transform steel into products (e.g., construction and automotive). For example, 30-50% of steel in automotive is currently wasted
- “Dirty” demolition scrap coming from end-of-life (EOL) goods, such as buildings, cars, and domestic appliances
Rates of scrap collection and usage are already high in regions with significant EAF penetration, such as Turkey (where it makes up 86% of production volumes), the US (79%), and the EU (43%). These figures are expected to increase with growth in EAF capacity from its current 14% of global steel production volumes (538 Mt annually of crude steel in 2022).
THE ECONOMIC ADVANTAGES OF SCRAP
As Figure 2 shows, using scrap is a highly competitive, efficient option for steel production compared to alternatives such as DRI powered by hydrogen or natural gas.
Taking the indexed cost of processing scrap steel at a baseline of 100, the costs for hydrogen DRI range from €130 to €164; for natural gas DRI, costs range from €93 to €158, depending on current and target-price scenarios. These figures demonstrate the economic feasibility of harnessing scrap steel, especially given that rising environmental regulations are expected to increase the cost of CO2 further.
GROWING GLOBAL DEMAND FOR SCRAP
The highest demand is for clean, new scrap. Arthur D. Little’s (ADL’s) model shows an annual shortage of 9 Mt in Europe by 2030, which will most likely push up prices. This means securing and improving “dirty” demolition scrap is crucial to plug the gap cost-effectively. This means that global competition for scrap is increasing, driving government and industry action across the world:
- Europe: Around 50 low-carbon steel projects are expected to be in place by 2030, requiring over 14 Mt of scrap in Europe alone and dramatically increasing competition for supplies. For example, to secure scrap supplies, ArcelorMittal has recently bought scrap metal recycling businesses in Poland, Germany, and the Netherlands. Since European players are currently consuming the majority of clean scrap produced in the region, they need to look further afield for additional supplies. At the same time, the European Parliament has approved a report that may create restrictions on scrap steel exports.
- US: American players are modernizing, with many new DRIs planned and M&A activities underway to secure scrap supply. For example, Sims Metal purchased Baltimore Scrap Corporation for US $177 million to better address the demand for scrap for green steel, adding 600 kilotons of capacity.
- China: China is currently structuring its scrap supply chain and seeing rapid growth in scrap use, which increased from 90 Mt in 2016 to 220 Mt in 2020. It is expected to reach ~400 Mt by 2030, driven by increasing green steel production investments. While currently behind other regions in scrap use because of low EAF penetration (under 15% of production), China is increasingly benefiting from access to growing volumes of EOL/demolition scrap.
- India: The Indian steel sector already has a scrap value chain, with imports growing by 63% between 2021 and 2022. It is expected to grow further to meet the needs of its developing steel producers, increasing by 50% by 2047.
- Africa: While considerable untapped scrap reserves exist in Africa, the scrap is difficult to collect and relies heavily on informal supply chains. Nevertheless, 1.8 Mt were exported in 2022, up from 1.1 Mt in 2020, showing the continent’s potential.
The necessary equipment for processing larger volumes of scrap steel is already in place, with many facilities currently possessing sufficient capacity. However, expanding scrap-processing operations requires significant capital investment in large storage facilities to accommodate higher volumes. These storage facilities should ideally be located closer to ports to enhance logistical efficiency and reduce transport costs. As a result, the industry faces substantial CAPEX requirements to build and maintain these expanded infrastructures, underscoring the financial challenges associated with scaling up scrap-processing capabilities.
5. DRIVING A NEED FOR NEW SKILLS AND CAPABILITIES
Over the past two decades, players in mature markets such as Europe have focused on extending the life and capacity of existing steelmaking plants through incremental investments. Green steel requires new skills — companies will need the capabilities to manage the construction, ramp-up, and operation of large-scale CAPEX projects built around new technologies. At the same time, they will need strategic and partnering skills to successfully manage energy sourcing and pricing (e.g., hydrogen versus gas for DRIs) across multiple time horizons. Global operators that have already implemented EAF/DRI plants will be at an advantage if they can transfer these skills to other regions, such as Europe.
Lessons from other sectors
As they seek to build circular economies around scrap steel, companies can learn from the experiences of other sectors, notably aluminum, glass, and urban mining.
Aluminum
Aluminum recycling saves up to 95% of the energy required for primary production, making it a cornerstone of the industry’s strategy to reduce carbon emissions. Companies such as Novelis, Constellium, and Arconic are leading the charge by investing heavily in advanced collection and recycling facilities that turn scrap aluminum into high-quality products.
Glass
By using cullet (recycled glass) in the production process, glass manufacturers reduce raw material consumption, lower energy use, and decrease CO2 emissions. For instance, using 1,000 kilograms (kg) of cullet saves approximately 580 kg of CO2 emissions compared to producing the same amount of glass from virgin materials.
Urban mining
Urban mining, the process of reclaiming raw materials from spent products, buildings, and waste, was pioneered in the electronics industry, where precious metals such as gold, silver, and copper are extracted from e-waste. It is now being extended to other areas, such as lithium-ion batteries. (See our article, “Opening the Urban Mine — Building a Profitable Circular Economy Based on a Lithium Battery Recycling Example” in this issue of Prism.) Urban mining alleviates the environmental degradation associated with traditional mining and reduces the strain on finite natural resources.
INSIGHTS FOR THE EXECUTIVE
Decarbonization is driving transformation in the steel industry, with the focus — especially in mature markets such as the EU and the US — shifting from optimizing existing production to large-scale CAPEX investments in new technologies such as DRI and EAF. For all those involved with steel as a producer or manufacturer of products, or in its recycling, the green transition and greater need for scrap bring opportunities. To ensure success, players should focus on three areas:
- Building ecosystems and fully circular economies to secure scrap supplies through partnerships and acquisitions across the supply chain.
- In constrained markets, looking globally for new, more cost-effective scrap sources, such as Africa and Latin America, and building new capabilities and partnerships to secure supplies.
- Developing new skills and capabilities to meet transforming market needs. This creates a greater need for competencies around managing complex supply chains to secure scrap supply, improving metallurgy and customer acceptance to absorb more scrap, building large-scale CAPEX projects from scratch to production, forecasting regulatory changes and impacts, and securing green energy supplies, as well as introducing new technologies — all while safeguarding quality and optimizing performance.
These lessons can be adapted for other circular economy–focused industries that are transforming to become more sustainable.
Notes
[1] According to IRENA (“Towards a Circular Steel Industry,” July 2023), steel production accounts for roughly 7% of global CO2 emissions.
[2] “Net-Zero Industry Tracker 2023.” World Economic Forum, 28 November 2023.
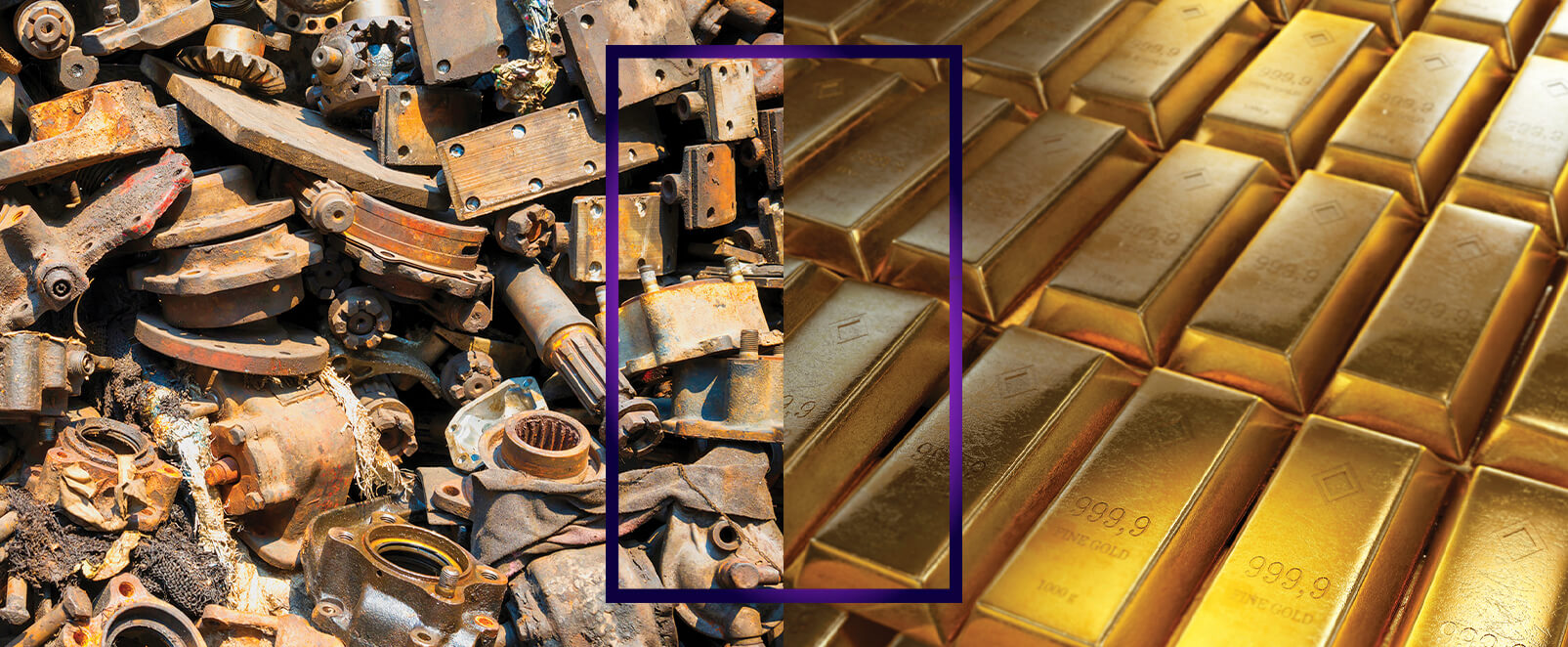
Is steel scrap the new gold?

DATE

Steel production is responsible for 7%[1] of global carbon dioxide (CO2) emissions, underscoring the urgency of reducing its carbon footprint to meet net zero targets. Regulators are intensifying their efforts; for instance, Europe aims to cut CO2 steel production emissions by nearly 25% by 2030.
Steel is integral to the global economy, playing a crucial role in the construction, automotive, and industrial machinery industries. It is also essential for green technologies such as wind turbines, electric vehicles, and advanced manufacturing processes. Despite volatility, steel demand is projected to rise, making its decarbonization pivotal for achieving a greener economy.
Two key technologies promise a more sustainable future for steel production: direct reduced iron (DRI) for primary steel and electric arc furnaces (EAFs) for secondary production, which reuse scrap steel. Deploying these green steel technologies is crucial for reducing emissions and replacing traditional, coal-fired blast furnaces.
However, successfully building and running DRI and EAF steel mills requires substantial investments, a supply of competitively priced green energy, and — the primary lever for EAF — acquiring sufficient scrap steel. In 2022, out of the 1,885 metric tons (Mt) of crude steel production, 1,340 Mt were produced using blast furnaces (around two tons of CO2 per ton of liquid metal), which should be replaced by DRI and EAF. EAF production was 538 Mt in the same period. This will drive large-scale demand growth in the scrap market. Scarcity will probably drive prices higher, particularly for clean scrap generated by manufacturing processes.
To secure their futures and meet the need for this vital material, steelmakers must transform to create more circular economies to secure and effectively use scrap. They need to move from digging in the ground for materials to digging in recycling sources. That requires closer collaboration with recyclers and manufacturers across the ecosystem that create scrap as a byproduct of their activities, such as automotive manufacturers, to collect sufficient volumes to power their new mills.
These changes are prompting steel producers to look globally for scrap, sourcing this new “gold” from Africa, China, Latin America, Europe, and North America. This expansion necessitates new strategies and operational models.
How can steel producers manage the green transition, and what are the lessons for other sectors as economies become more sustainable and circular?
MEETING THE GLOBAL IMPERATIVE TO DECARBONIZE STEEL
REGULATORY PRESSURES
Traditional steel production methods involve blast furnaces that use coking coal as fuel and inevitably produce large amounts of CO2. Around 85% of steel production was powered by fossil fuels in 2022, according to the World Economic Forum.[2] While efforts are ongoing to reduce or capture emissions, such as by carbon capture and storage (CCS), large-scale deployment remains limited because of economic, technical, and infrastructural challenges. Many projects are still at the proof-of-concept stage, such as ArcelorMittal’s Steelanol Project in Belgium, which aims to capture CO2 from steel production and convert it into ethanol through a biological process.
Meanwhile, the regulatory clock is ticking. Annual emissions from steel production represent 5% of the EU’s total CO2. This has led EU regulators to target reducing emissions from 191 Mt in 2021 to 150 Mt by 2030. Individual firms have also set their own targets; for example, US Steel aims to reduce greenhouse gas emissions intensity by 20% by 2030 and achieve net zero emissions by 2050. Regulatory change is being driven by a combination of targets, carbon taxes (including the EU’s Carbon Border Adjustment Mechanism [CBAM], which aims to ensure imported materials such as steel are produced sustainably), and national/international subsidies for the switch to cleaner technologies such as DRI and EAF.
CUSTOMER PRESSURES
Major customers are also increasingly demanding low-emission steel, driven by their own sustainability targets. For example, leading US automotive manufacturers such as Ford and General Motors have joined the First Movers Coalition, a global group committed to decarbonizing supply chains, including steel.
THE IMPORTANCE OF TECHNOLOGY INNOVATION
Together, these regulatory and customer pressures are driving a need for change in the steel industry, which is manifested in a shift away from emissions-heavy blast furnaces to cleaner technologies that rely on three elements:
- Green energy
- Hydrogen
- Sufficient supplies of scrap
DRI AND EAF
DRI furnaces replace the coking coal traditionally used in the iron ore reduction process with gas or hydrogen to produce directly reduced (liquid) iron. This is then fed into an EAF, along with scrap steel, at a ratio of at least 50% scrap. This combination is melted to form liquid steel. It is then cast and rolled to transform the steel into coils or plates.
Emissions are considerably reduced, particularly if green feedstock (such as green hydrogen) replaces coking coal and the electricity used within the EAF is from renewable sources. However, the cost of producing liquid metal will increase without access to cheap, decarbonized energy to power these processes.
Demonstrating the potential of this green steel, steel producer SSAB, iron ore miner LKAB, and energy company Vattenfall collaborated to create HYBRIT (Hydrogen Breakthrough Ironmaking Technology), a fossil-free iron and steelmaking technology. In June 2021, the three companies showcased the world’s first hydrogen-reduced sponge iron, which was then used to produce steel provided to Volvo. Volume production by SSAB is scheduled to begin in 2026.
THE CHALLENGES TO THE PRODUCTION OF GREEN STEEL
Five challenges face the steel industry as it aims to transition from old-style blast furnaces to cleaner DRI-EAF steel production: technological maturity, growing CAPEX/OPEX investment, securing green energy, securing sufficient scrap, and the need for new skills/capabilities.
1. TECHNOLOGY MATURITY
EAF technology is fully mature and already makes up a substantial proportion of global steel production volumes. It is currently being extended to make new steel types. However, it requires a combination of scrap and primary steel from DRI plants, replacing inputs from blast furnaces. Yet, DRI technology is currently at the developmental stage, with many existing installations relying on natural gas rather than green fuels such as hydrogen. While significant investments have been announced in DRI (over €10 billion in Europe alone), no DRI facilities of 2.5 Mt that consume hydrogen have yet been built. Therefore, scaling green DRI pilots into mature installations is critical to reducing emissions and ensuring a green future for steel.
2. GROWING CAPEX AND OPEX INVESTMENT
In mature markets, many previous investments focused on process improvements or increasing capacity/quality. Business cases were, therefore, relatively simple to calculate based on projected market demand. Securing investment in green steel projects is much more complex, as it requires assumptions on CO2 prices, energy costs, and planned regulations/carbon taxes. According to Eurofer, meeting the EU’s 2030 targets requires producers to invest an estimated €85 billion — €31 billion in CAPEX and €54 billion in OPEX — of which €15-17 billion has been announced. This is leading to heavy reliance on state subsidies to fund new plants around the world, including:
- €850 million from France to ArcelorMittal
- €2 billion from the German state to ThyssenKrupp
- £500 million from the UK government to Tata Steel
- US $500 million each from the US Department of Energy to steelmakers Cleveland-Cliffs and SSAB for green steel plants
3. SECURING GREEN ENERGY
Producers must source a continuous green energy supply for DRI and EAF facilities. Consequently, replacing blast furnaces with DRI-EAF means energy will make up a much higher percentage of running costs than iron ore based on current market conditions. Securing these energy supplies at competitive prices is vital for success:
- Green and blue hydrogen for DRI: Competition is growing for (currently limited) green and blue hydrogen supplies, which require renewable energy for production and carbon capture technology, respectively. A significant reduction in the price of hydrogen is necessary to make DRI cost competitive.
- Green electricity for EAF: Given the need for constant electricity availability to power EAFs, hydroelectric and nuclear power are the most appropriate sources, rather than intermittent solar or wind. However, the availability of such sources varies considerably by country and region, again pushing up costs and handing advantages to producers located near such energy sources.
Recently announced European DRI and EAF projects are estimated to require 60 gigawatt hours (GWh) of green energy annually, covering both the direct supply of green electricity and the green energy required to produce hydrogen through electrolysis.
Investments are also being made in new sites that benefit from access to renewable energy and green hydrogen. Vulcan Green Steel (VGS) has begun construction of a greenfield steel complex in Duqm, Oman. When production begins in 2027, it will run on 100% renewable wind and solar energy and incorporate green hydrogen once it becomes available.
4. SECURING SUFFICIENT SCRAP
Scrap steel is essential for EAFs, in which it is melted alongside liquid iron from DRI. It comes from three main sources (see Figure 1):
- Internal “clean” steel mill scrap that is traditionally consumed by the producer
- Clean scrap from downstream players that transform steel into products (e.g., construction and automotive). For example, 30-50% of steel in automotive is currently wasted
- “Dirty” demolition scrap coming from end-of-life (EOL) goods, such as buildings, cars, and domestic appliances
Rates of scrap collection and usage are already high in regions with significant EAF penetration, such as Turkey (where it makes up 86% of production volumes), the US (79%), and the EU (43%). These figures are expected to increase with growth in EAF capacity from its current 14% of global steel production volumes (538 Mt annually of crude steel in 2022).
THE ECONOMIC ADVANTAGES OF SCRAP
As Figure 2 shows, using scrap is a highly competitive, efficient option for steel production compared to alternatives such as DRI powered by hydrogen or natural gas.
Taking the indexed cost of processing scrap steel at a baseline of 100, the costs for hydrogen DRI range from €130 to €164; for natural gas DRI, costs range from €93 to €158, depending on current and target-price scenarios. These figures demonstrate the economic feasibility of harnessing scrap steel, especially given that rising environmental regulations are expected to increase the cost of CO2 further.
GROWING GLOBAL DEMAND FOR SCRAP
The highest demand is for clean, new scrap. Arthur D. Little’s (ADL’s) model shows an annual shortage of 9 Mt in Europe by 2030, which will most likely push up prices. This means securing and improving “dirty” demolition scrap is crucial to plug the gap cost-effectively. This means that global competition for scrap is increasing, driving government and industry action across the world:
- Europe: Around 50 low-carbon steel projects are expected to be in place by 2030, requiring over 14 Mt of scrap in Europe alone and dramatically increasing competition for supplies. For example, to secure scrap supplies, ArcelorMittal has recently bought scrap metal recycling businesses in Poland, Germany, and the Netherlands. Since European players are currently consuming the majority of clean scrap produced in the region, they need to look further afield for additional supplies. At the same time, the European Parliament has approved a report that may create restrictions on scrap steel exports.
- US: American players are modernizing, with many new DRIs planned and M&A activities underway to secure scrap supply. For example, Sims Metal purchased Baltimore Scrap Corporation for US $177 million to better address the demand for scrap for green steel, adding 600 kilotons of capacity.
- China: China is currently structuring its scrap supply chain and seeing rapid growth in scrap use, which increased from 90 Mt in 2016 to 220 Mt in 2020. It is expected to reach ~400 Mt by 2030, driven by increasing green steel production investments. While currently behind other regions in scrap use because of low EAF penetration (under 15% of production), China is increasingly benefiting from access to growing volumes of EOL/demolition scrap.
- India: The Indian steel sector already has a scrap value chain, with imports growing by 63% between 2021 and 2022. It is expected to grow further to meet the needs of its developing steel producers, increasing by 50% by 2047.
- Africa: While considerable untapped scrap reserves exist in Africa, the scrap is difficult to collect and relies heavily on informal supply chains. Nevertheless, 1.8 Mt were exported in 2022, up from 1.1 Mt in 2020, showing the continent’s potential.
The necessary equipment for processing larger volumes of scrap steel is already in place, with many facilities currently possessing sufficient capacity. However, expanding scrap-processing operations requires significant capital investment in large storage facilities to accommodate higher volumes. These storage facilities should ideally be located closer to ports to enhance logistical efficiency and reduce transport costs. As a result, the industry faces substantial CAPEX requirements to build and maintain these expanded infrastructures, underscoring the financial challenges associated with scaling up scrap-processing capabilities.
5. DRIVING A NEED FOR NEW SKILLS AND CAPABILITIES
Over the past two decades, players in mature markets such as Europe have focused on extending the life and capacity of existing steelmaking plants through incremental investments. Green steel requires new skills — companies will need the capabilities to manage the construction, ramp-up, and operation of large-scale CAPEX projects built around new technologies. At the same time, they will need strategic and partnering skills to successfully manage energy sourcing and pricing (e.g., hydrogen versus gas for DRIs) across multiple time horizons. Global operators that have already implemented EAF/DRI plants will be at an advantage if they can transfer these skills to other regions, such as Europe.
Lessons from other sectors
As they seek to build circular economies around scrap steel, companies can learn from the experiences of other sectors, notably aluminum, glass, and urban mining.
Aluminum
Aluminum recycling saves up to 95% of the energy required for primary production, making it a cornerstone of the industry’s strategy to reduce carbon emissions. Companies such as Novelis, Constellium, and Arconic are leading the charge by investing heavily in advanced collection and recycling facilities that turn scrap aluminum into high-quality products.
Glass
By using cullet (recycled glass) in the production process, glass manufacturers reduce raw material consumption, lower energy use, and decrease CO2 emissions. For instance, using 1,000 kilograms (kg) of cullet saves approximately 580 kg of CO2 emissions compared to producing the same amount of glass from virgin materials.
Urban mining
Urban mining, the process of reclaiming raw materials from spent products, buildings, and waste, was pioneered in the electronics industry, where precious metals such as gold, silver, and copper are extracted from e-waste. It is now being extended to other areas, such as lithium-ion batteries. (See our article, “Opening the Urban Mine — Building a Profitable Circular Economy Based on a Lithium Battery Recycling Example” in this issue of Prism.) Urban mining alleviates the environmental degradation associated with traditional mining and reduces the strain on finite natural resources.
INSIGHTS FOR THE EXECUTIVE
Decarbonization is driving transformation in the steel industry, with the focus — especially in mature markets such as the EU and the US — shifting from optimizing existing production to large-scale CAPEX investments in new technologies such as DRI and EAF. For all those involved with steel as a producer or manufacturer of products, or in its recycling, the green transition and greater need for scrap bring opportunities. To ensure success, players should focus on three areas:
- Building ecosystems and fully circular economies to secure scrap supplies through partnerships and acquisitions across the supply chain.
- In constrained markets, looking globally for new, more cost-effective scrap sources, such as Africa and Latin America, and building new capabilities and partnerships to secure supplies.
- Developing new skills and capabilities to meet transforming market needs. This creates a greater need for competencies around managing complex supply chains to secure scrap supply, improving metallurgy and customer acceptance to absorb more scrap, building large-scale CAPEX projects from scratch to production, forecasting regulatory changes and impacts, and securing green energy supplies, as well as introducing new technologies — all while safeguarding quality and optimizing performance.
These lessons can be adapted for other circular economy–focused industries that are transforming to become more sustainable.
Notes
[1] According to IRENA (“Towards a Circular Steel Industry,” July 2023), steel production accounts for roughly 7% of global CO2 emissions.
[2] “Net-Zero Industry Tracker 2023.” World Economic Forum, 28 November 2023.